Table of Contents
Introduction
Hormones and it’s effects on crop growth represent one of the most powerful yet underutilized tools in modern agriculture. These chemical messengers orchestrate virtually every aspect of plant development, from seed germination to fruit ripening, and understanding their complex interactions can transform agricultural productivity. Whether you’re a commercial farmer seeking to boost yields or a researcher exploring sustainable farming methods, mastering plant hormone applications provides a competitive edge in an increasingly challenging growing environment.
Importance
Plant hormones, also called phytohormones, are naturally occurring organic compounds that regulate growth, development, and responses to environmental stimuli. These powerful chemical messengers act as signaling molecules that coordinate complex physiological processes within crop plants. What makes plant hormones particularly fascinating is their ability to cause dramatically different effects depending on:
- The specific plant species
- The developmental stage of the plant
- The concentration of the hormone
- The presence of other hormones (synergistic or antagonistic interactions)
- Environmental conditions
The importance of hormones and their effects on crop plants can’t be overstated in modern agriculture. They enable farmers to manipulate plant growth cycles, enhance stress resistance, improve fruit quality, and ultimately increase marketable yields.
Historical Development of Plant Hormones Research
The journey to understand hormones and their effects on crop plants began in the late 19th century when Charles Darwin and his son Francis conducted experiments on phototropism—the bending of plants toward light. Their work, published in “The Power of Movement in Plants” (1880), suggested that an “influence” transmitted from the tip of grass seedlings controlled their bending toward light.
This “influence” was later identified as auxin, the first discovered plant hormone. The timeline of major discoveries includes:
Decade | Key Developments |
1930s | Isolation and characterization of auxin (indole-3-acetic acid or IAA) |
1940s | Discovery of gibberellins in Japan through research on “foolish seedling disease” in rice |
1950s | Identification of cytokinins and their role in cell division |
1960s | Characterization of abscisic acid and its functions in dormancy and stress responses |
1970s | Recognition of ethylene as a gaseous hormone affecting ripening |
1990s-2000s | Discovery of brassinosteroids, jasmonates, salicylic acid, and strigolactones |
2010s-present | Advanced understanding of hormone signaling pathways and receptors |
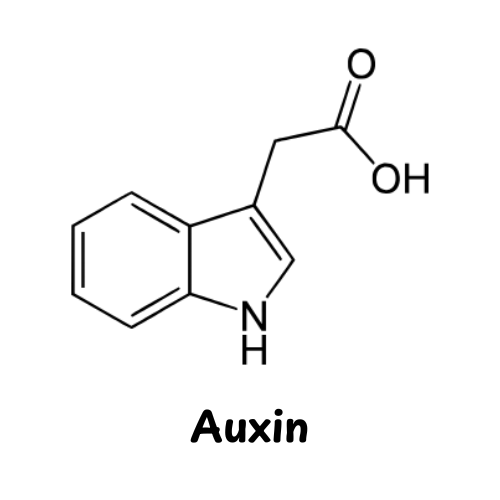
Auxins: The Growth Regulators
When discussing hormones and their effects on crop plants, auxins often take center stage as the quintessential growth regulators. These versatile compounds influence virtually every aspect of plant development, from cell elongation to root initiation, making them invaluable tools for modern agriculture.
Natural vs. Synthetic Auxins
Natural auxins, primarily indole-3-acetic acid (IAA), are produced in the apical meristems of shoots and transported downward through the plant in a polar fashion. This creates concentration gradients that drive many developmental processes. In contrast, synthetic auxins like 2,4-D, NAA (naphthaleneacetic acid), and IBA (indolebutyric acid) have been developed to mimic the effects of natural auxins, often with enhanced stability and specificity.
The comparative advantages of synthetic auxins include:
- Longer persistence in plant tissues
- Greater resistance to enzymatic breakdown
- More specific action on target tissues
- Easier application and commercial availability
- Cost-effectiveness for large-scale agricultural use
However, natural auxins remain essential to the plant’s intrinsic regulatory systems and often work in complex feedback loops with other hormones that synthetic versions may disrupt.
Effects on Root Development
The relationship between auxins and root development exemplifies the concentration-dependent nature of hormones and their effects on crop plants. At optimal concentrations (typically 10^-8 to 10^-6 molar), auxins stimulate:
- Initiation of lateral roots from the pericycle
- Formation of adventitious roots on stems or leaves
- Extension of the primary root system
- Development of root hairs that increase absorption surface area
This root-promoting property is harnessed commercially in rooting powders and gels containing auxins like IBA, which dramatically improve success rates when propagating plants from cuttings.
Interestingly, at higher concentrations, auxins inhibit root elongation while promoting lateral root formation—a response that can be exploited when a bushier, more fibrous root system is desired. This apparent contradiction illustrates the complexity of hormones and their effects on crop plants at different concentrations.
Gibberellins: Stem Elongation and Seed Germination
Among the various hormones and their effects on crop plants, gibberellins (GAs) stand out for their dramatic influence on plant stature and reproductive transitions. First identified through research on the “foolish seedling disease” that caused rice plants to grow abnormally tall and topple over, GAs have since become essential tools in modern crop management.
Applications in Breaking Seed Dormancy
One of the most commercially significant aspects of hormones and their effects on crop plants involves gibberellin’s role in seed dormancy. GA applications can:
- Stimulate germination in dormant seeds by activating hydrolytic enzymes
- Replace natural cold stratification requirements
- Synchronize germination across a seed lot
- Overcome physiological dormancy mechanisms
For crop producers, this translates to practical advantages like:
- Uniform emergence: GA-treated seeds often germinate more synchronously, making subsequent field operations more efficient.
- Extended growing seasons: Breaking dormancy artificially allows earlier planting or production in regions with insufficient chilling periods.
- Improved seedling vigor: Properly timed GA treatments can enhance early growth rates and establishment.
- Higher germination percentages: Especially valuable for species with naturally low or erratic germination rates.
Increasing Fruit Size with Gibberellins
Beyond their effects on vegetative growth and germination, gibberellins significantly influence reproductive development, particularly fruit growth. Commercially, GA applications can:
- Increase berry size in seedless grape varieties
- Promote fruit set in apple orchards during adverse pollination conditions
- Delay rind aging in citrus fruits
- Replace pollination requirements in some parthenocarpic fruits
However, gibberellin applications come with important considerations. Excessive use can reduce return flowering the following season in perennial crops, illustrating how hormones and their effects on crop plants must be balanced against long-term productivity goals.
Cytokinins: Cell Division Masters
Within the complex interplay of hormones and their effects on crop plants, cytokinins serve as the primary regulators of cell division and differentiation. These nitrogen-containing compounds, structurally similar to adenine, play critical roles in numerous developmental processes that directly impact agricultural productivity.
Cytokinin Roles in Lateral Bud Development
The phenomenon of apical dominance—where the main growing shoot suppresses the development of lateral buds—exemplifies the antagonistic relationship between auxins and cytokinins. This interplay of hormones and their effects on crop plants allows for architectural manipulation through:
- Exogenous cytokinin applications to stimulate lateral branching
- Pruning techniques that alter endogenous hormone balances
- Breeding for altered cytokinin sensitivity or transport
Delaying Senescence with Cytokinins
Perhaps the most visibly dramatic effect of cytokinins is their ability to delay senescence—the programmed aging and death of plant tissues. This “anti-aging” property has significant implications for postharvest management and ornamental longevity.
Practical applications exploiting these effects of hormones on crop plants include:
- Extending the shelf life of leafy vegetables through postharvest cytokinin dips
- Prolonging the vase life of cut flowers with cytokinin-containing preservatives
- Maintaining green color in turfgrass during stress periods with cytokinin-based biostimulants
- Delaying leaf yellowing in potted plants with foliar cytokinin sprays
The molecular mechanisms behind cytokinin-delayed senescence involve:
- Preservation of chlorophyll by inhibiting chlorophyllase activity
- Maintained protein synthesis and reduced proteolytic enzyme activity
- Enhanced antioxidant defense systems
- Continued nutrient import into treated tissues
Abscisic Acid: The Stress Hormone
Among the various hormones and their effects on crop plants, abscisic acid (ABA) stands out for its central role in stress responses and resource allocation during challenging conditions. Unlike growth-promoting hormones, ABA often acts as a “brake” on developmental processes, helping plants survive adverse environments by inducing appropriate physiological adaptations.
ABA’s Role in Seed Dormancy
Beyond stress responses, ABA plays a crucial role in seed development and dormancy—another vital aspect of hormones and their effects on crop plants. During seed maturation, ABA:
- Promotes the accumulation of storage proteins and lipids
- Induces desiccation tolerance mechanisms
- Suppresses premature germination (vivipary)
- Establishes dormancy states that prevent germination under unfavorable conditions
The agricultural implications of these effects are significant. Insufficient ABA during seed development can lead to pre-harvest sprouting in cereals, particularly during wet harvest conditions, resulting in substantial quality and economic losses. Conversely, excessive dormancy can cause uneven germination and poor crop establishment.
Ethylene: Ripening and Senescence
Among the major plant hormones and their effects on crop plants, ethylene holds a unique position as the only gaseous hormone and a key regulator of ripening and senescence processes. Its commercial significance is immense, influencing postharvest quality, shelf life, and market timing for numerous fruit and vegetable crops.
Ethylene Inhibitors in Postharvest Management
The discovery and development of ethylene action inhibitors has revolutionized fresh produce handling, providing powerful tools to manipulate hormones and their effects on crop plants throughout distribution networks.
Environmental Factors Affecting Ethylene Production
The production and action of ethylene illustrate how environment interacts with hormones and their effects on crop plants. Key environmental factors influencing ethylene metabolism include:
Environmental Factor | Effect on Ethylene | Agricultural Implications |
Temperature | Higher temperatures generally increase ethylene production up to 30°C, then enzymes denature | Cooling is essential for postharvest management; temperature management critical during ripening |
Physical damage | Wounding dramatically increases ethylene synthesis | Careful handling required; damaged produce accelerates ripening of undamaged items |
Oxygen levels | Low O₂ reduces ethylene production and sensitivity | Controlled atmosphere storage exploits this relationship |
Carbon dioxide | Elevated CO₂ competes with ethylene for receptor binding | CO₂ treatments can slow ripening responses |
Water stress | Moderate stress often increases ethylene production | Drought can accelerate senescence; irrigation management affects shelf life |
Pathogen attack | Infection sites produce significant ethylene | One bad apple really does spoil the bunch through ethylene transfer |
Future Directions in Hormonal Management of Crops
The study of hormones and their effects on crop plants continues to evolve rapidly, with several emerging trends likely to shape agricultural practices in coming years. As climate challenges intensify and resource efficiency becomes increasingly critical, sophisticated hormonal management approaches offer promising pathways to sustainable intensification.
Integration of artificial intelligence with plant hormone research represents a particularly exciting frontier. Machine learning algorithms analyzing thousands of hormone-response scenarios across diverse environments can identify patterns invisible to traditional research approaches. These insights will likely enable increasingly precise recommendations for specific crop-environment combinations, moving beyond generalized protocols toward truly customized hormonal management strategies.
Similarly, advances in phenomics—the large-scale study of plant physical
and biochemical traits—are increasingly linked to hormonal profiles. High-throughput phenotyping platforms now capable of monitoring subtle growth responses can be correlated with endogenous hormone levels or responses to exogenous applications. This integration of hormones and their effects on crop plants with digital agriculture technologies promises more evidence-based intervention strategies.
Looking forward, several key developments will likely characterize the future of plant hormone applications:
- Climate-adaptive hormonal strategies: As growing conditions become less predictable, hormonal interventions will increasingly focus on building resilience rather than simply maximizing yields under ideal conditions. This might include:
- Priming treatments that prepare crops for anticipated stresses
- Hormonal applications timed according to real-time weather prediction models
- Integration with climate-smart agriculture approaches
- Hormone-microbiome interactions: Growing recognition of how soil and plant microbiomes influence, produce, and metabolize plant hormones will lead to integrated management systems that:
- Leverage beneficial microorganisms as hormone delivery systems
- Select microbial inoculants based on their hormonal effects
- Consider impacts of agricultural practices on hormone-related microbial functions
- Consumer-focused applications: As consumer preferences increasingly drive agricultural priorities, hormonal management will expand beyond productivity to target specific quality traits:
- Enhanced nutritional profiles through targeted hormonal interventions
- Improved flavor development in fruits and vegetables
- Extended shelf life with minimal intervention
- Reduced environmental impacts through greater precision
- Hormonal approaches to novel growing systems: Urban agriculture, vertical farming, and other controlled environment systems will increasingly utilize precise hormonal management to:
- Optimize space utilization through architectural manipulation
- Accelerate crop cycles in controlled environments
- Synchronize production with market demands
- Create entirely new production systems and opportunities
These advances in understanding and applying hormones and their effects on crop plants will require integrated approaches that combine fundamental research with practical field applications. Success will depend on bridging traditional disciplinary boundaries between molecular biology, crop physiology, agricultural engineering, and farming practice.
Ultimately, the future of hormonal management in agriculture lies not in viewing these compounds as simple growth enhancers or problem-solvers, but as sophisticated tools within integrated crop management systems. When properly understood and thoughtfully applied, plant hormones offer some of our most powerful approaches to creating agricultural systems that are simultaneously productive, resilient, and sustainable—addressing the complex challenges facing global food production in the decades ahead.
By developing a deeper understanding of hormones and their effects on crop plants across scales from molecular to field levels, agricultural scientists and practitioners can unlock new possibilities for feeding the world while preserving the natural resources upon which all agriculture depends.
Frequently Asked Questions About Plant Hormones
What are the main differences between natural and synthetic plant hormones?
Natural plant hormones are compounds produced endogenously by plants to regulate their growth and development. Synthetic plant hormones, also called plant growth regulators (PGRs), are man-made chemicals designed to mimic or interfere with natural hormone functions. The key differences include:
- Stability: Synthetic hormones typically remain active longer in plant tissues as they resist enzymatic breakdown.
- Specificity: Many synthetic hormones target specific receptors or pathways more precisely than their natural counterparts.
- Application: Natural hormones function within intricate feedback systems, while synthetic versions can be applied externally at specific times and concentrations.
- Cost and availability: Synthetic hormones are generally more economical for commercial applications and more readily available in standardized formulations.
Understanding these distinctions helps growers make informed decisions about using hormones and their effects on crop plants in different agricultural contexts.
How do environmental stresses affect hormone balance in crops?
Environmental stresses significantly alter the delicate balance of hormones and their effects on crop plants. Major stress-induced hormonal changes include:
- Drought stress: Increases ABA production, which closes stomata and activates water conservation mechanisms while often reducing cytokinin and gibberellin levels, slowing growth.
- Temperature extremes: Heat stress can increase ethylene production, accelerating senescence, while cold stress may elevate ABA and reduce gibberellin levels, slowing metabolic processes.
- Salinity: Triggers ABA accumulation similar to drought responses while disrupting cytokinin transport from roots to shoots.
- Nutrient deficiencies: Often reduce cytokinin synthesis (particularly under nitrogen limitation) and alter auxin transport patterns.
- Light stress: Excessive light can trigger jasmonate and salicylic acid production associated with defense responses.
These stress-induced hormonal shifts underlie many visible symptoms of plant stress and represent adaptive mechanisms to prioritize survival over growth or reproduction under challenging conditions.
Can organic farmers use hormonal treatments on their crops?
Organic farmers have limited but important options for influencing hormones and their effects on crop plants within organic certification standards. Approved approaches include:
- Natural plant extracts: Seaweed extracts containing natural cytokinins and auxins are widely permitted in organic systems.
- Microbial inoculants: Many beneficial microorganisms produce plant hormones or hormone-like compounds that influence plant development.
- Plant-derived compounds: Certain botanical preparations containing natural plant hormones are approved for specific uses.
- Cultural practices that influence endogenous hormone balance:
- Pruning to alter auxin distribution
- Cover cropping with allelopathic species that release hormone-like compounds
- Timing of operations to coincide with natural hormonal cycles
Notably, synthetic hormones and growth regulators like 2,4-D, NAA, and gibberellic acid are generally prohibited in certified organic production, though specific exemptions exist for limited applications in some organic standards.
What are the risks of hormone overuse in agriculture?
While hormones and their effects on crop plants offer valuable management tools, their overuse can lead to several problems:
- Phytotoxicity: Excessive hormone applications can damage plant tissues, causing leaf curling, epinasty, or abnormal growth patterns.
- Reduced return flowering: Some hormones, particularly gibberellins, can suppress flower bud formation for the following season when overused on perennial crops.
- Altered source-sink relationships: Disrupting natural hormone balances can change how plants allocate resources, sometimes at the expense of root development or storage organ formation.
- Environmental concerns: Some synthetic auxins can drift and damage sensitive neighboring crops; breakdown products may affect soil microbial communities.
- Development of tolerance: Repeated use of certain hormone treatments may lead to diminished plant responses over time.
- Unpredictable interactions: Excessive application of one hormone can disrupt multiple hormonal pathways due to complex crosstalk mechanisms.
Best practices involve using the minimum effective dose, proper timing based on plant phenology, and integrating hormonal treatments with other management approaches rather than relying on them exclusively.
How do plant hormones influence crop quality beyond yield?
Hormones and their effects on crop plants extend well beyond simple yield increases to influence numerous quality parameters important to consumers and processors:
- Nutritional composition: Cytokinins can increase protein content in some cereals; ABA influences antioxidant accumulation in fruits under moderate stress.
- Flavor compounds: Ethylene coordinating ripening processes affects volatile production and sugar-acid balances crucial to flavor development.
- Texture characteristics: Auxins and gibberellins influence cell wall composition and structure, determining firmness, juiciness, and processing characteristics.
- Visual appeal: Hormonal balance affects chlorophyll retention, anthocyanin development, and uniform coloration—key factors in market acceptance.
- Storage potential: Pre-harvest hormone management can significantly influence postharvest respiration rates, susceptibility to disorders, and overall shelf life.
- Processing suitability: For processing crops, hormones can alter dry matter content, pectin composition, and other factors affecting processing efficiency.
These quality impacts make hormones and their effects on crop plants increasingly important in high-value horticultural chains where quality often determines profitability more than absolute yield.
What emerging technologies are changing how we use plant hormones?
Innovation continues to transform applications of hormones and their effects on crop plants. Cutting-edge approaches include:
- Nanotechnology delivery systems: Encapsulating hormones in biodegradable nanoparticles for:
- Slow, controlled release
- Reduced environmental exposure
- Lower required application rates
- Target-specific delivery to particular plant tissues
- Gene editing for hormone pathway modification:
- CRISPR-Cas9 techniques targeting specific hormone biosynthesis or signaling genes
- Creating crops with optimized hormonal profiles for specific environments
- Developing semi-dwarf varieties with altered gibberellin responses
- Computational modeling of hormone interactions:
- Systems biology approaches to predict complex hormonal crosstalk
- Decision support tools for optimal application timing
- Predictive phenotyping based on hormonal profiles
- Hormone-responsive biosensors:
- Real-time monitoring of plant hormone status in field conditions
- Integration with precision agriculture systems
- Early stress detection through hormonal signatures
These technologies promise more precise, efficient, and environmentally sound applications of knowledge about hormones and their effects on crop plants, potentially revolutionizing how we manage crop development in coming decades.